First observation of gravitational waves from merging neutron stars
Discovery marks first cosmic event observed in both gravitational waves and light
For the first time, astronomers have observed the gravitational waves – ripples in the fabric of space-time – and the light from the merger of two neutron stars. The event observed on August 17th 2017, at 12:41:04 UTC, marks the advent of multi-messenger astronomy which combines gravitational-wave and electromagnetic observations. Together, the complementary methods will enhance our understanding of extreme astrophysical events, and provide an unprecedented opportunity to probe the outcome of the collision of two neutron stars. Researchers at the Max Planck Institute for Gravitational Physics (Albert Einstein Institute/AEI) in Potsdam and Hannover and at the Leibniz Universität Hannover played a central role in the discovery.
The discovery, named GW170817, was made using the U.S.-based Laser Interferometer Gravitational-Wave Observatory (LIGO), the Europe-based Virgo detector, and about 70 ground- and space-based astronomical observatories that detected the electromagnetic signal emitted by the neutron star collision. Neutron stars are compact remnants from supernova explosions and consist of extremely dense matter. They measure about 20 kilometers across and have up to two times as much mass as our Sun, or almost 700,000 times as much mass as our Earth.
A revolutionary view of merging neutron stars
“This first detection of merging neutron stars through gravitational waves is extremely exciting in itself, but its combination with dozens of electromagnetic follow-up observations makes it truly revolutionary,” say Bruce Allen and Alessandra Buonanno, directors at the Max Planck Institute for Gravitational Physics, and Karsten Danzmann, director at the Max Planck Institute for Gravitational Physics and at the Institute for Gravitational Physics of the Leibniz Universität Hannover. “The identification of GW170817 with a binary system composed of two neutron stars and the observations of electromagnetic radiation after the collision shed light on the so far mysterious origin of short gamma-ray bursts.”
The results are published today in the journal Physical Review Letters. Further papers from the LIGO and Virgo collaborations and other astronomical communities have been either submitted or accepted for publication in various journals.
Pinpointing the source of the waves
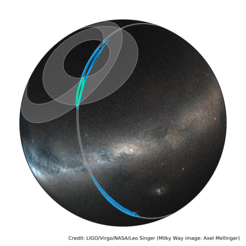
GW170817 was observed by both LIGO instruments for about 100 seconds on August 17th, 2017. The Virgo instrument significantly improved the sky localization, allowing researchers to better pinpoint the source in a patch of the sky of only 28 square degrees (about 130 times the apparent size of the full moon) in the southern celestial hemisphere.
Just 1.7 seconds later, the Gamma-ray Burst Monitor on the Fermi space telescope detected a burst of gamma rays (GRB 170817A) from about the same direction as the gravitational-wave signal. The chance coincidence of the gamma-ray burst and gravitational wave was calculated to be extremely small, so these two events unambiguously share the same progenitor.
The very precise localization provided by the LIGO-Virgo detection made it possible for a handful of observatories around the world, hours later, to start searching the region of the sky where the signal was thought to originate. A new point of light, resembling a new star, was first found by optical telescopes near the galaxy NGC 4993. Ultimately, about 70 observatories on the ground and in space observed the event at their representative wavelengths, including X-ray, ultraviolet, optical, infrared, and radio waves.
These new observations reveal signatures of recently synthesized material, including gold and platinum, solving a decades-long mystery of where about half of all elements heavier than iron are produced.
Deforming neutron stars
Analysis of the LIGO data placed the neutron star merger at the relatively close distance of about 85 to 160 million light-years from Earth, in agreement with the measured distance of 130 million light-years to the suspected host galaxy NGC 4993. In contrast to previous gravitational-wave observations, the masses of the merging objects were estimated to be 1.1 to 1.6 times that of our Sun, similar to that of known neutron stars and incompatible with black holes.
How matter behaves in neutron stars is not well known and described by their unknown “equation of state” or internal structure. Depending on this equation the extremely dense matter is more or less stiff when deformed. Studying the imprint of the deformations in the gravitational-wave signal caused by mutual tidal forces just before the merger, scientists were able to show that certain proposed equations of state are unlikely to be true.
Measuring the expansion of the Universe with gravitational waves
Previous work by Bernard Schutz, director emeritus of the AEI Potsdam, showed that gravitational-wave signals from merging neutron stars can be used to determine the Hubble constant in a new and independent way. By combining the distance obtained from the gravitational-wave observation with the measurement of the cosmological redshift from electromagnetic observations, the expansion of the Universe can be observed independently of previously used methods. The association with NGC 4993 allows a new kind of independent measurement of the Hubble constant, which agrees with previous results. Future observations of similar events will improve this measurement.
Observing and identifying GW170817: A first look inside a neutron star
GW170817: Numerical relativity simulation of a binary neutron star merger
To observe minute-long binary-neutron-star signals buried in the noise and to characterize them requires very accurate waveform models. Members of the “Astrophysical and Cosmological Relativity” division at the AEI in Potsdam have developed waveform models that were directly used as templates in the matched-filter-based searches that found GW170817. Max Planck scientists also played a leading role in building and running the search algorithms that observed GW170817. As part of the rapid-response team to GW170817, they promptly removed a loud non-Gaussian transient present in the data recorded by the LIGO-Livingston observatory. This allowed to pinpoint the sky-location accurately enough so that astronomers were able to observe, within 12 hours of GW170817’s detection, the fast-fading optical afterglow and to identify the host galaxy, and redshift, which led to the measurement of the Hubble constant.
Max Planck scientists used analysis codes and waveform models they co-developed to identify the source of GW170817 as a binary composed of two neutron stars, finding that theories that predict strongly repulsive nuclear forces – which result in comparatively large and therefore easily distorted stars – are disfavored. Analytical calculations and numerical-relativity simulations pursued in Potsdam were further used to construct state-of-the-art waveform models that predicted weaker repulsive nuclear forces in the component stars of the GW170817 merger. Max Planck scientists also studied possible electromagnetic signals produced by the matter ejected during GW170817’s merger. Those signatures can unfold the formation of heavy elements in our Universe.
“As a domino effect, GW170817 set off one of the most spectacular sequence of astrophysical events observed in astronomy, solving a longstanding enigma, but also opening novel inquiries”, says Alessandra Buonanno, director at the AEI in Potsdam and College Park Professor at the University of Maryland. “Remarkably, GW170817 also allowed us to shed the first light on the nature of superdense matter at the core of the most fascinating and extreme objects in our Universe: neutron stars.”
Max Planck scientists in Potsdam are currently carrying out analyses to test the consistency of GW170817 with a binary neutron-star signal as predicted by General Relativity.
Finding and studying GW170817 from the first hour
First LIGO/Virgo detection of a binary neutron star merger (GW170817)
Members of the “Observational Relativity and Cosmology” division at the AEI in Hannover played a central role in the first hours of the analysis, the characterization of the gravitational-wave signal, and in understanding the nature of the source.
“I was completely wrong. Even in my wildest dreams I never thought our first double neutron star gravitational wave detection would have a coincident gamma ray burst and electromagnetic counterparts. I thought we would see something like this only after twenty or more double neutron star mergers had been observed, not with the very first one. It's fantastic!”, says Bruce Allen, director at the AEI Hannover.
AEI Hannover researchers together with AEI Potsdam and other LIGO researchers in the rapid-response team excised an instrumental artifact in data of one LIGO detector and used software developed in Hannover to help generate the first precise sky map. This led to the detection of the optical afterglow of the neutron star merger within hours of the gravitational-wave detection and enabled the determination of the host galaxy, its redshift, and an independent measurement of the Hubble constant.
AEI Hannover scientists also led and contributed significantly to the “offline analysis” of the three-detector network that identified GW170817 with high statistical significance. They served on the detection committee and contributed to understanding how the very small signal in Virgo data was consistent with the sky location of the source and the detector sensitivity.
The scientists developed and contributed to the only waveform models that can be used to test for misaligned neutron star spins and to models that account for tidal effects in neutron stars, which were used to understand the behavior of neutron star matter. They provided numerical simulations of the merger, and studied possible fates of the remnant.
The division operates Atlas, the most powerful computer cluster in the world designed for gravitational-wave searches and detailed follow-up analyses of signals. Atlas contributed 58 million CPU cores hours to the analysis of LIGO/Virgo data from the second observation run.
“Observational Relativity and Cosmology” researchers also contributed to several of the accompanying papers.
Developing and testing crucial detector technology with GEO600
GEO600 from above
The GEO Collaboration, a team of Max Planck, Leibniz Universität Hannover and UK researchers, has been operating the GEO600 gravitational-wave detector south of Hannover, Germany, since the mid 1990s. GEO600 is a development center for novel and advanced technologies in the international gravitational-wave research community.
Many key technologies that enable the unprecedented sensitivity of LIGO and its discoveries have been developed and tested at GEO600. AEI researchers together with the Laser Zentrum Hannover e.V. also developed, built, and installed the high-power laser systems at the heart of the LIGO instruments. Crucial improvements in the optical measurement principle such as combined power and signal recycling were first demonstrated at high sensitivity in GEO600. It is the only gravitational-wave detector worldwide using “squeezed light”, which in the future all ground-based gravitational-wave detectors will employ to further improve their sensitivity.
GEO600 data are also used in a search for the post-merger remnant, such as a hyper-massive neutron star. The detector’s good sensitivity at high frequencies means that it would be well suited to pick up the temporary rapid vibrations emitted by such an object.
“This is the beginning of multi-messenger astronomy and of a deeper understanding of our Universe. We are very proud to play a central role in the detection of gravitational waves because our lasers – that were developed and tested in the GEO600 project – are at the heart of all gravitational wave observatories“, says Karsten Danzmann, director at the AEI in Hannover and at the Institute for Gravitational Physics of the Leibniz Universität Hannover.
LIGO and Virgo
LIGO is funded by the NSF, and operated by Caltech and MIT, which conceived of LIGO and led the Initial and Advanced LIGO projects. Financial support for the Advanced LIGO project was led by the NSF with Germany (Max Planck Society), the U.K. (Science and Technology Facilities Council) and Australia (Australian Research Council) making significant commitments and contributions to the project. More than 1,200 scientists and some 100 institutions from around the world participate in the effort through the LIGO Scientific Collaboration, which includes the GEO Collaboration and the Australian collaboration OzGrav. Additional partners are listed at http://ligo.org/partners.php.
The Virgo collaboration consists of more than 280 physicists and engineers belonging to 20 different European research groups: six from Centre National de la Recherche Scientifique (CNRS) in France; eight from the Istituto Nazionale di Fisica Nucleare (INFN) in Italy; two in the Netherlands with Nikhef; the MTA Wigner RCP in Hungary; the POLGRAW group in Poland; Spain with the University of Valencia; and the European Gravitational Observatory, EGO, the laboratory hosting the Virgo detector near Pisa in Italy, funded by CNRS, INFN, and Nikhef.